Morgan & Masterson Consulting
Biological Safety and Risk Analyses
Biological Safety and Risk Analyses
Most regulatory authorities require evidence of biological safety of medical devices, such evidence being provided by successful completion of standard test procedures, which are directed towards satisfactory performance related to a series of biological end points. These end points may include cytotoxicity, sensitization, acute and chronic toxicity, thrombogenicity, mutagenicity, carcinogenicity and reproductive toxicity. The precise tests that are used for such determinations are not usually mandated by regulators, but it is usual, and indeed sensible, to follow the procedures recommended by the International Standards Organization (ISO) in their ISO 10993 series. The selection of tests to be used for any new submission is not normally problematic and there are many commercial test houses that can advise on this, and carry out such tests under GLP conditions. However, there are some contentious issues, where a much deeper knowledge of biocompatibility and toxicology is required, some of which are covered here.
Interpretation of ISO 10993
Questions also arise when there is no adequate test to determine performance with respect to certain endpoints. Two important biological responses may be mentioned, those of carcinogenicity and thrombogenicity. The former refers to the possibility that biomaterials could be the cause of cancer. Most regulators require complete investigations of mutagenicity testing as a type of surrogate for carcinogenicity but, from a biological perspective it is possible for non- mutagenic substances to give rise to cancer, so there are residual concerns. The problem is that there are no reliable animal models for implant-induced tumor formation – when I carried out animal experiments in my Liverpool laboratories. I could produce tumors with all currently used biomaterials in rats. The justification of claims that biomaterials are not carcinogenic requires careful crafting. With respect to thrombogenicity, there are standard in vitro tests for effects on the blood clotting cascades and on platelet function, but their relevance to intravascular devices is far from clear, and all test data has to be assessed by those experienced in the field.
Chemical characterization and toxicology
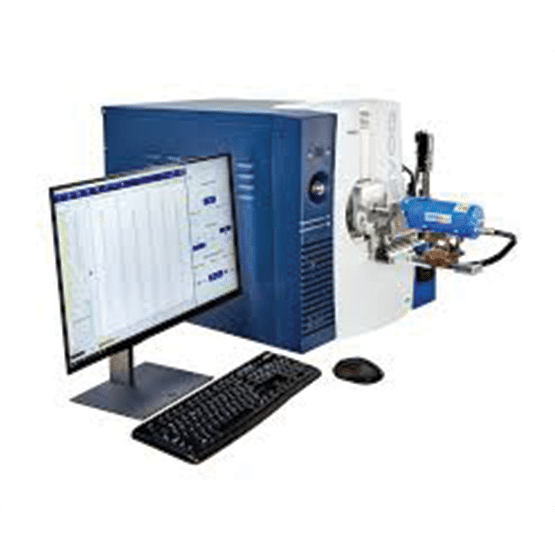
Gap analysis
Failure analysis
On rare occasions, the investigation has to be more profound than determining fault and settling claims for damages. This is usually because there may be many patients (perhaps world-wide) who have been implanted with a device that is now being shown to be associated with a ‘defect’, which could cause death or injury on a significant scale. I have been involved with several such scenarios. In one case, which I can mention since this was long ago and all claims settled, a mechanical heart valve was shown to fail in a few patients after several years function. By the time this was realized, over 80,000 patients had received the valve. The critical question concerned the decisions as to whether such patients should have their valves surgically removed and replaced with another prosthetic valve. A group was established, approved by the Board of the company and the FDA, with responsibility of determining the characteristics of those patients who should be considered for re-operation. Of significance was the fact that statistically there was an overall risk of death at reoperation of 6%; the risk of valve fracture overall could not be assessed since this was a time-dependent fatigue process. As a metallurgist, my role was to determine the precise mechanism of failure and identify those valves (size, date and place of manufacturer, the name of the welders and other manufacturing personnel etc.) most at risk, while other team members, who were biostatisticians, epidemiologists, cardiac surgeons and so on, determined the patients most at risk. My report was very detailed, and has never been made public, but I was very satisfied with the outcome of that consulting experience.
Morgan & Masterson Consulting
We offer global, high-level consulting for scientific and infrastructure aspects of cutting-edge medical technologies.
Our Expertise
Work with Us
We are commited to making a global impact and providing cost-effective solutions.