A Focus on Innovative Solutions and Strategic Decision-Making
We faced significant challenges when founding Strait Access Technologies, some being of geo- political, regulatory and health economic nature, but mostly centered around the technology platform that would deliver our aspirational goal. Some aspects of the technology were being used elsewhere, but not at the same time or within this particular RHD patient population. Some of these challenges needed highly innovative solutions.
It was initially decided that we would direct the main effort to the replacement of diseased aortic valves (in preference to the other three type of heart valve) and that the target pathology was regurgitation rather than stenosis. It was recognized that, in view of limited resources, it was better to target regurgitant aortic valves, such that if the new technology was successful there, it could possibly be directed to other conditions in the future. It was further decided to aim for the transapical route and to use a balloon-expandable system, as mentioned in the section on RHD.
Navigating Regulatory Challenges and Innovative Engineering in South African TAVR Development
The developing regulatory system in South Africa was also relevant. The South African Health Products Regulatory Authority (SAHPRA) only came into existence after the founding of SAT and there was some hesitancy about how this body would respond to a submission related to a complex implantable medical device that was reliant on some as-yet unproven clinical technologies. Partly because of this, we decided to work on a valve that used natural tissues for the leaflets, since this type of material was successfully used in products in developed countries. The ideal SAT valve would have leaflets made of a durable polymer, but it was considered sensible to get proof of concept with the tissue valve before attempting clinical use with the polymer. Even then, it would not necessarily be an easy approval process since the tissue valves ultimately degrade, and this was more likely in the younger RHD patients than in the usual elderly patient with aortic stenosis.
The major engineering innovations concerned the delivery systems that would allow placement of the TAVR within the heart. There were two options for the delivery route, one using the more popular access via the femoral artery, and one using an approach that involves a direct route via a small incision in the apex of the left ventricle of the heart. We chose the latter technique as being more appropriate for the RHD patients and aimed to develop an innovative balloon expansion system, which would be more adaptable for health facilities with less sophisticated imaging systems.
The Valve Expansion Mechanism
The SAT technology platform was, therefore taking shape. The delivery system and expansion mechanism were central features of the platform. Concerning the expansion mechanism, we were well aware that most TAVR designs and systems, world-wide, favored self-expansion rather than balloon expansion products. However, the shape-memory nitinol stents used in the former type actually expand rapidly, so that accurate placement is at a premium, necessitating sophisticated imaging facilities and, in many cases, the need for rapid pacing of the heart during deployment to prevent valve dislodgement. We therefore designed a non-occlusive helical balloon, which incorporates self-locating retractable balloon trunks that facilitate easy positioning and placement within the aortic root; the invaginating retrieval principle makes it impossible to be pinched between the stent and the aortic wall (see three different perspectives in the Figure). The balloon expansion concept allows the device to reach a pre-determined constant diameter, exerting constant controlled radial force.
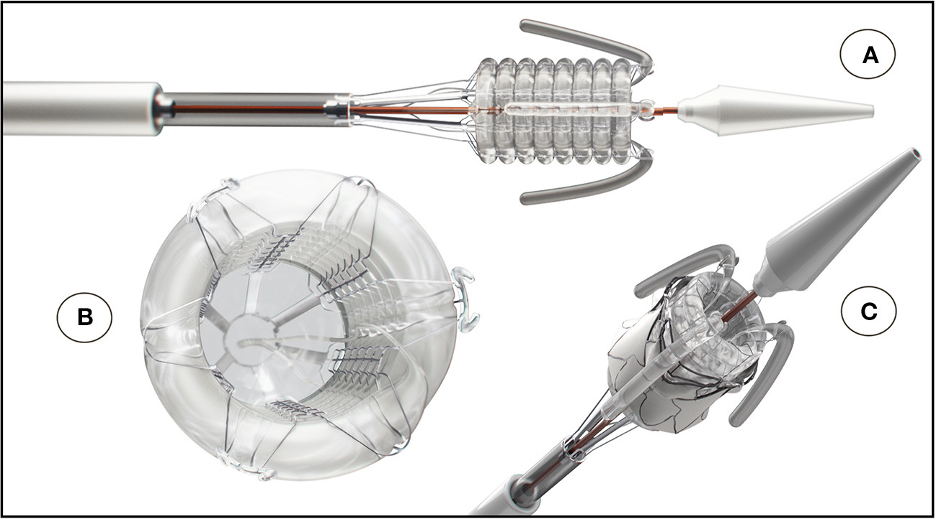
The Valve Materials
A balloon expandable TAVR is constructed with flexible leaflets (usually three to mimic the natural aortic valve) which are mounted on a metal framework. As noted above, we agreed that our first product would be a bioprosthetic valve, that is with leaflets made of animal tissues, since, generically, these had a good record of performance in both surgical and transcatheter replacements, and offered, in principle, a more straightforward regulatory pathway. Our bioprosthetic valve is made of bovine pericardium, the sac-like structure that envelops the heart and roots of major blood vessels in cows. The sourcing is important since there can be no risks of infectivity. A couple of decades ago, the presence of spongiform encephalopathies in some species, especially cows (giving rise to the BSE phenomenon) led to a restriction on the use of tissues from such animals in medical devices; the major risk management process has been the sourcing of tissues from countries that were certified BSE-free, which determined that we would have to source tissues from cattle in Namibia, the only African country internationally recognized as BSE- free.
The pericardial sacs from freshly killed cows are collected from our chosen abattoir and cleaned, one sac being retained for DNA and histological analysis, the remainder being stored in sterile distilled water and then transferred to a decellularization solution with an antibacterial preservative and refrigerated. Many different chemicals and protocols have been used by other companies to minimize the risks of immunogenicity, infectivity and calcification.
These protocols are critical to the use of the heart valve prostheses, so a vast amount of development and testing has been undertaken in our Cape Town laboratories in order to optimize our procedures.
The frame on which the leaflets are attached is made of a highly corrosion resistant cobalt- nickel- chromium alloy known as MP35N, widely used in other implantable medical devices. Both the leaflets and frame, usually referred to as a stent, have computer-generated designs. The precision geometry of the stent is achieved by laser cutting. The leaflets are stitched onto the frame by trained operators, which is a time-consuming process. A fibrous polymeric skirt is attached to the bottom part of the stent to avoid backflow of blood.
In the photographs, the manufactured stent is shown on the left. The stent has to be collapsed to a size capable of being contained with the delivery catheter; this process is known as crimping, the crimped stent being shown in the center image. Once inside the annulus of the aortic root, the stent is expanded to the final dimensions (right image), where it anchors to the tissue of that annulus. One part of the innovative design of the valve is the mechanism by which this anchorage takes place. All of these innovations have full patent protection. It should be appreciated that these images show just the stent component; the leaflet structure is contained within the stent, sutured through the holes visible in the scalloped members. We have valves to fit either 26 or 29 mm annulus, the crimped structure obviously being much smaller.
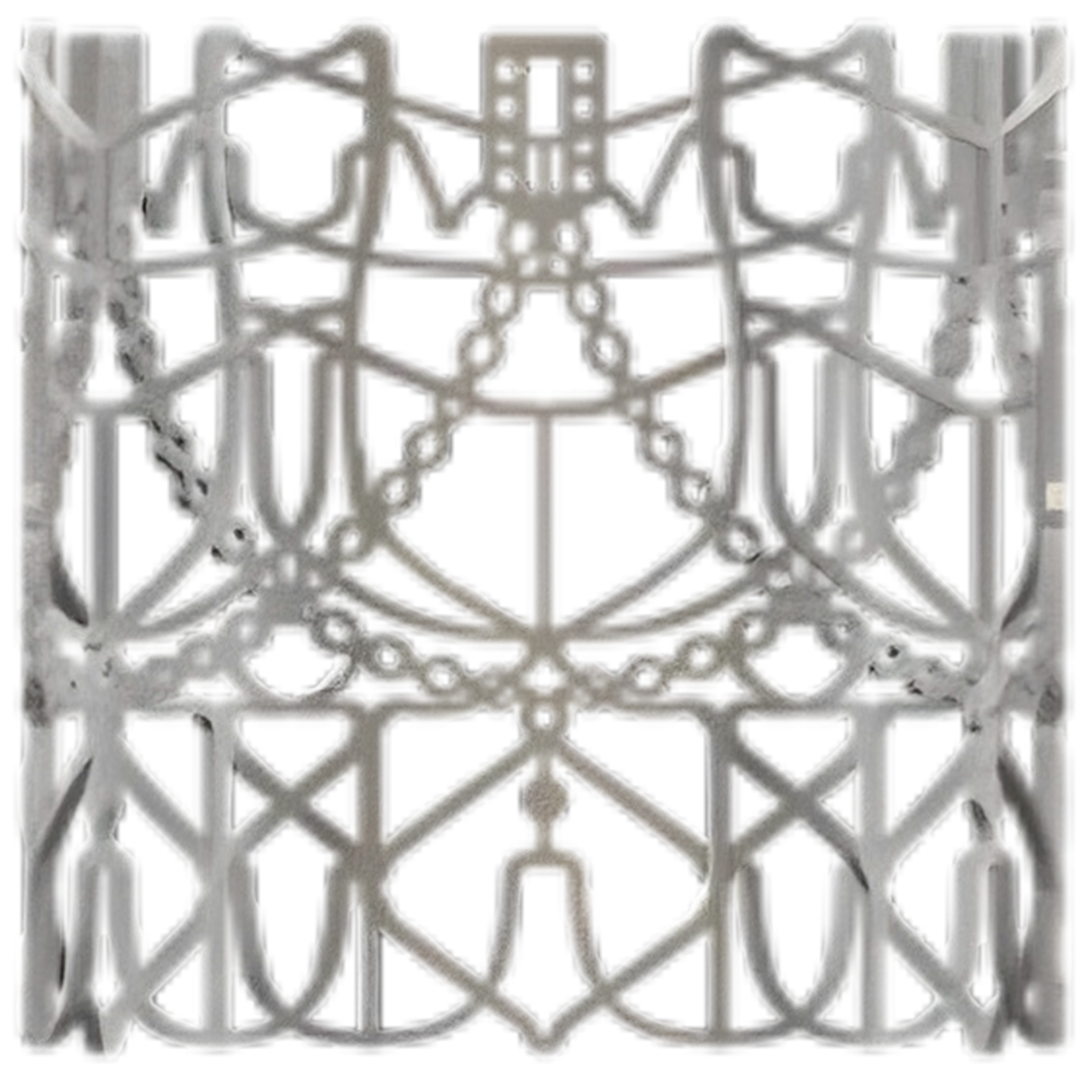
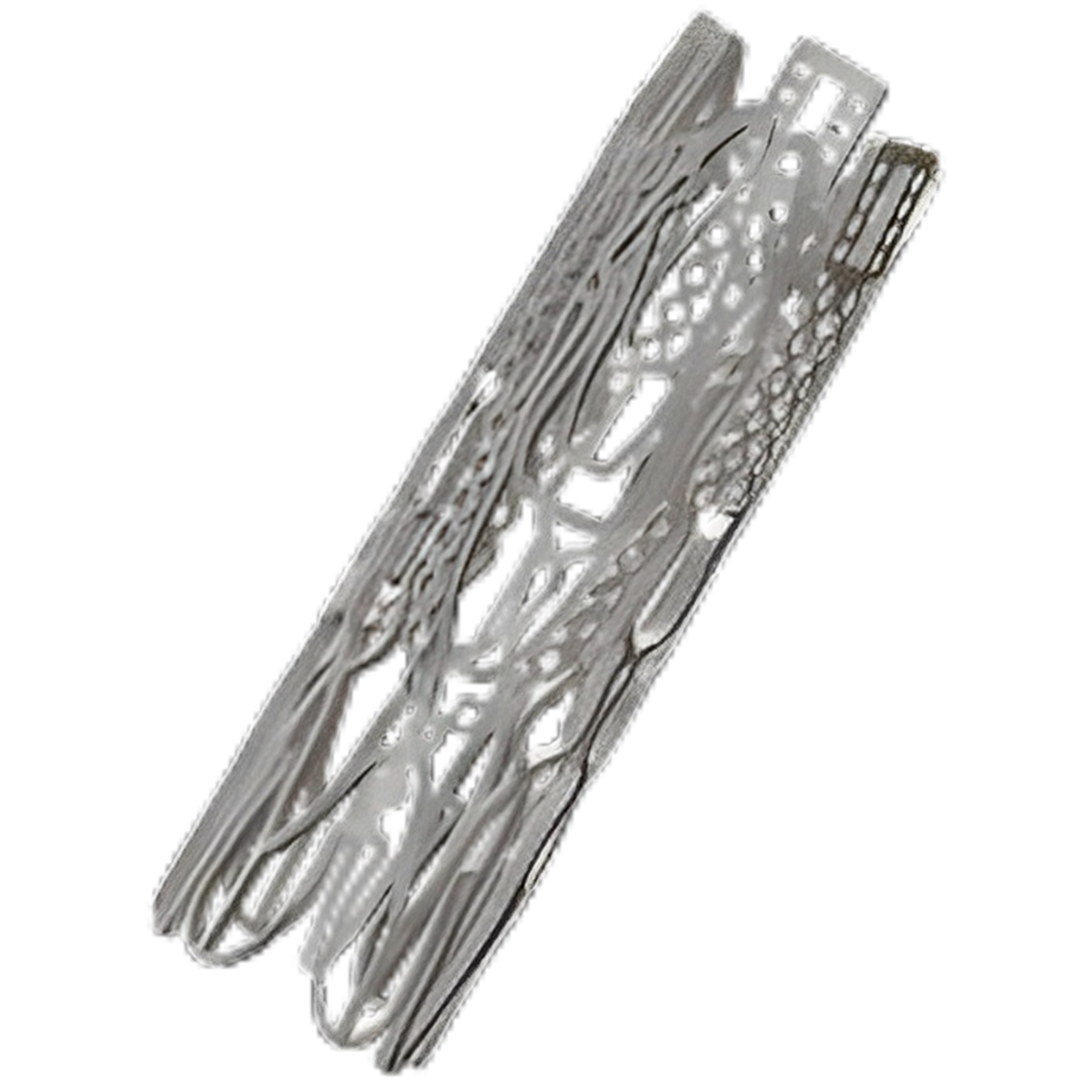
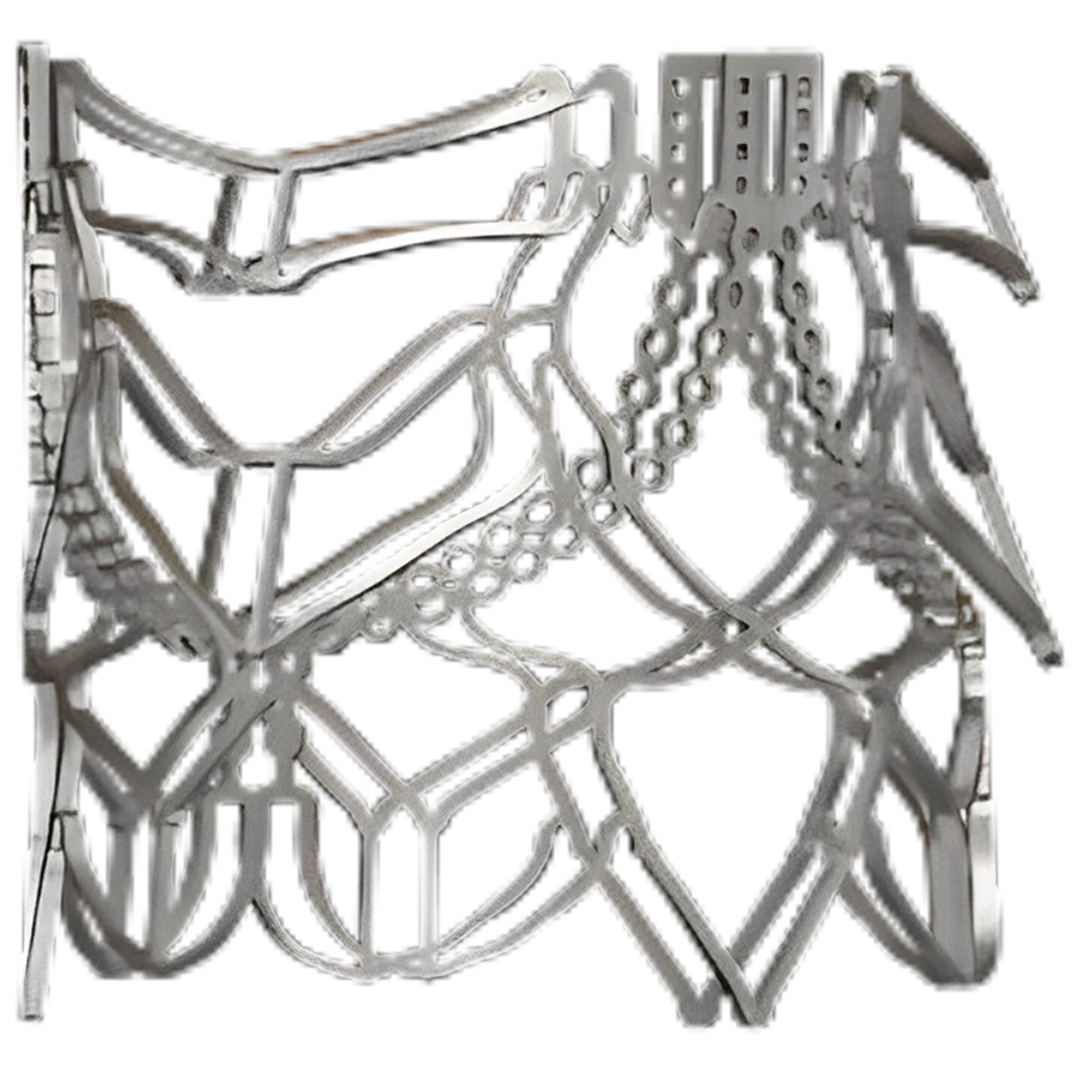
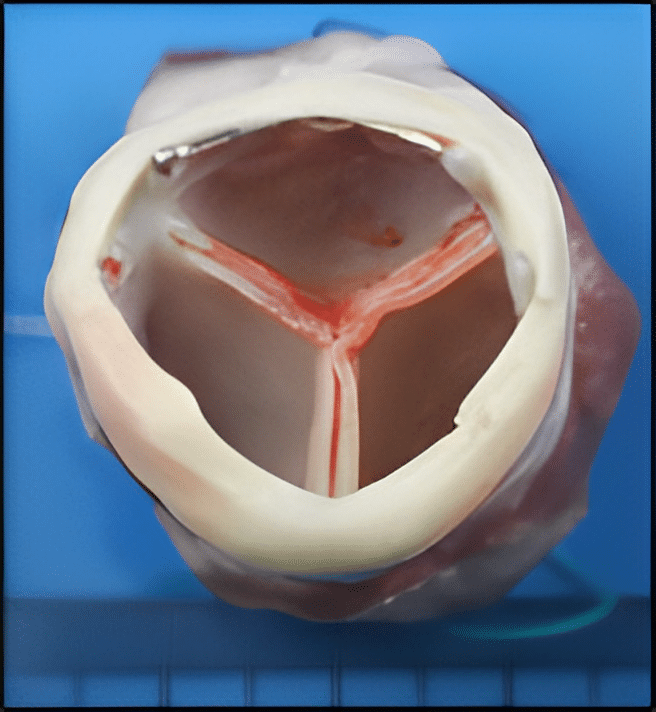
A SAT Bioprosthetic TAVR explanted from an animal, showing fully healed tissues, is shown in the diagram.
Before permission can be obtained for first use in human, our valves have had to undergo very rigorous testing. This involves extensive evaluation of blood flow characteristics through the valve in laboratory pulse duplicators and fatigue testing of the structures to ensure that they can endure 400 million opening and closing cycles without any evidence of structural change in either the stent or the leaflets. Pulse duplicator and fatigue facilities are shown in the images:
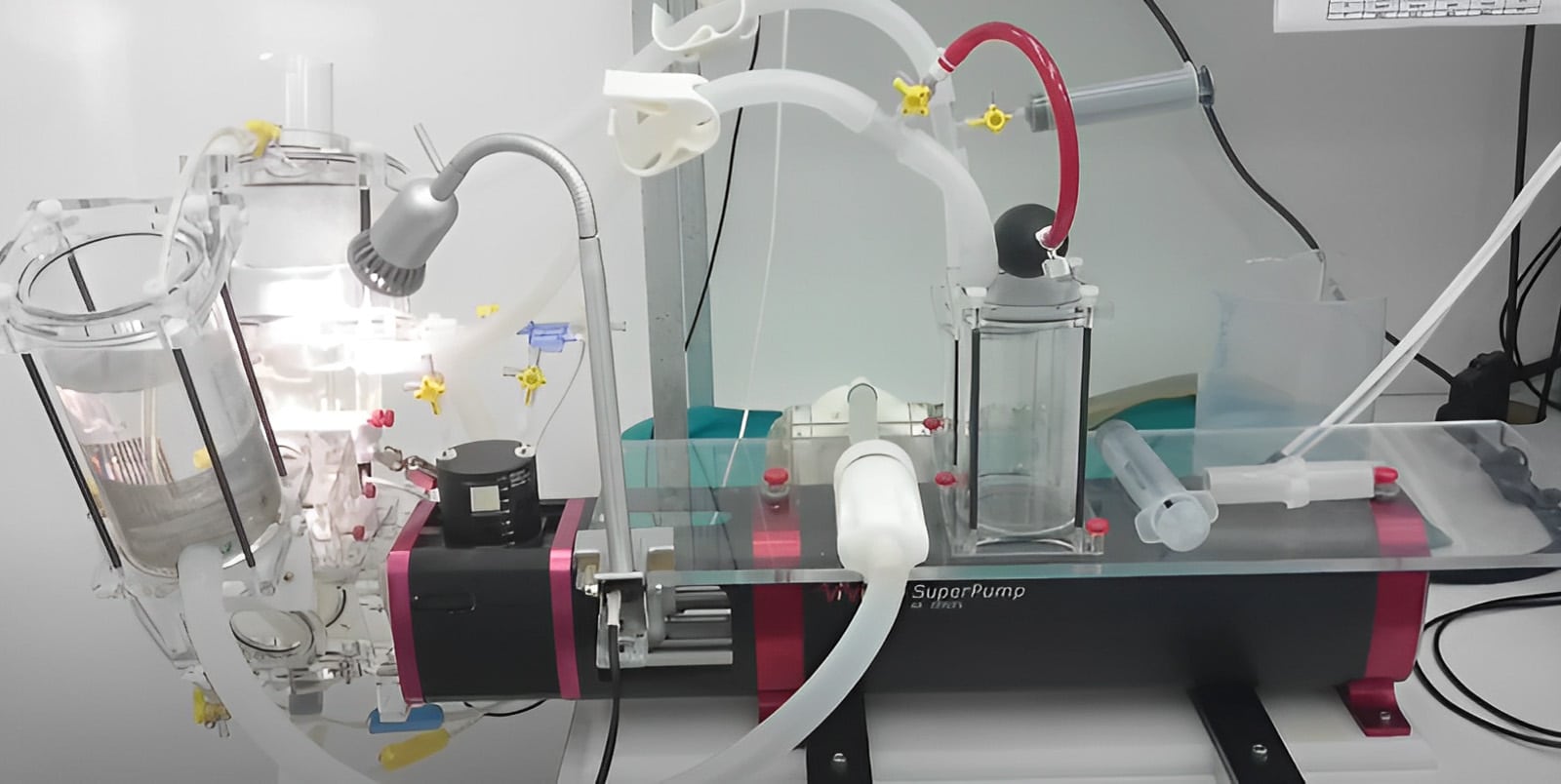
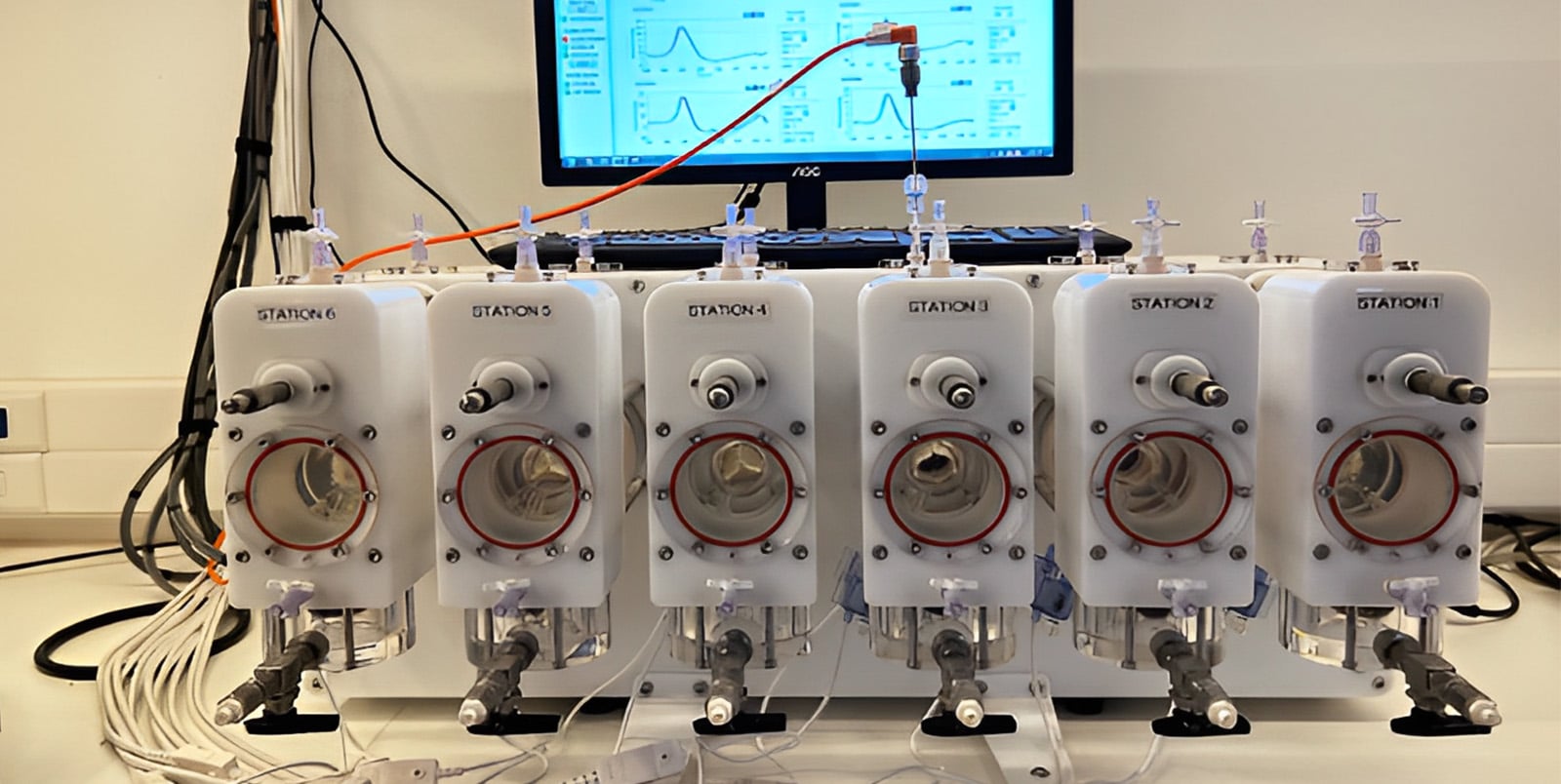
Following successful laboratory tests, it was then necessary for us to evaluate out valve in large animal models. International standards mandate the broad principles under which tests are carried out. They require up to five months implantation in either sheep or pigs.
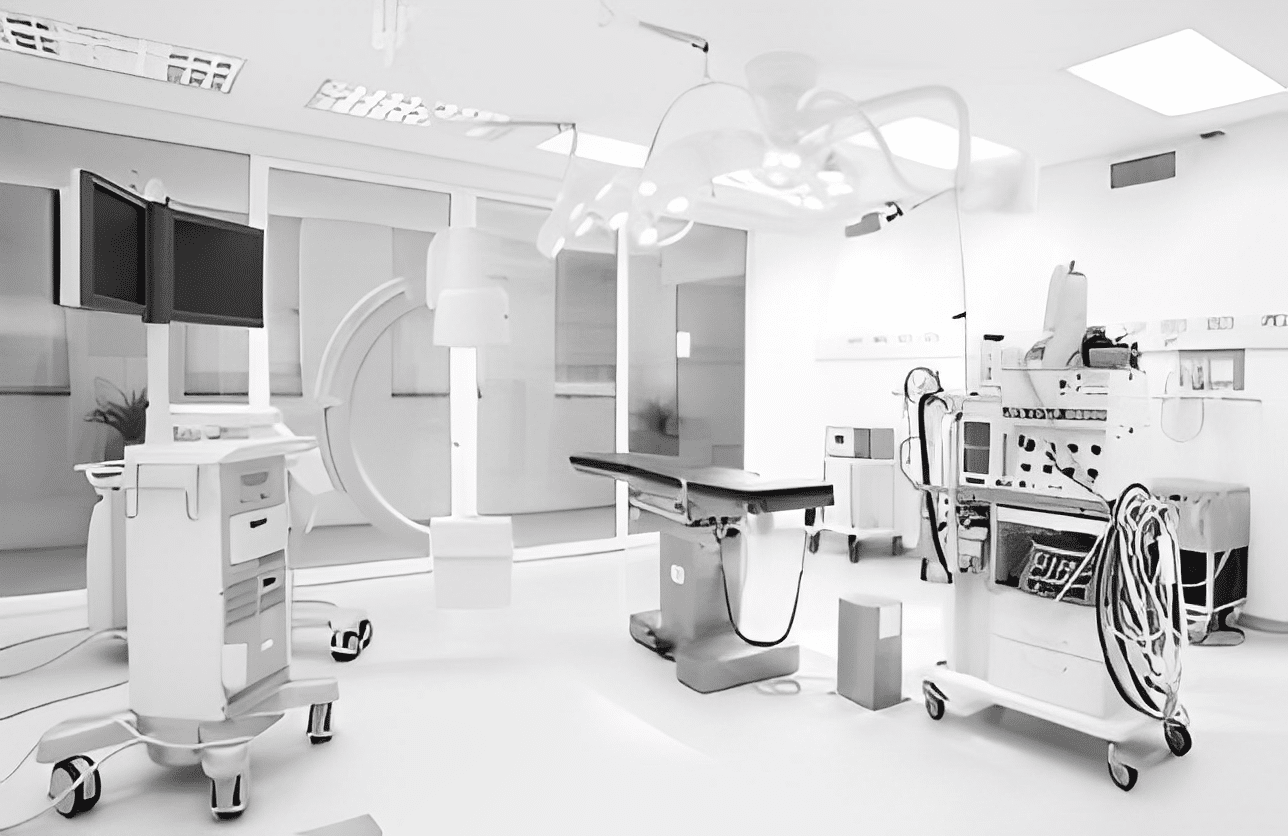
We have been able to use the extensive animal facilities in the University of Cape Town for this work.
The outline of our technology platform described in this section has led to the development of several product prototypes, including this bioprosthetic TAVR, but also, now, a polymeric equivalent, a separate dilatation balloon and a balloon product that will assist in the implantation of stent grafts to treat aortic aneurysms.